Exploring Hidden Variables in Quantum Physics: Can They Explain Everything?
Written on
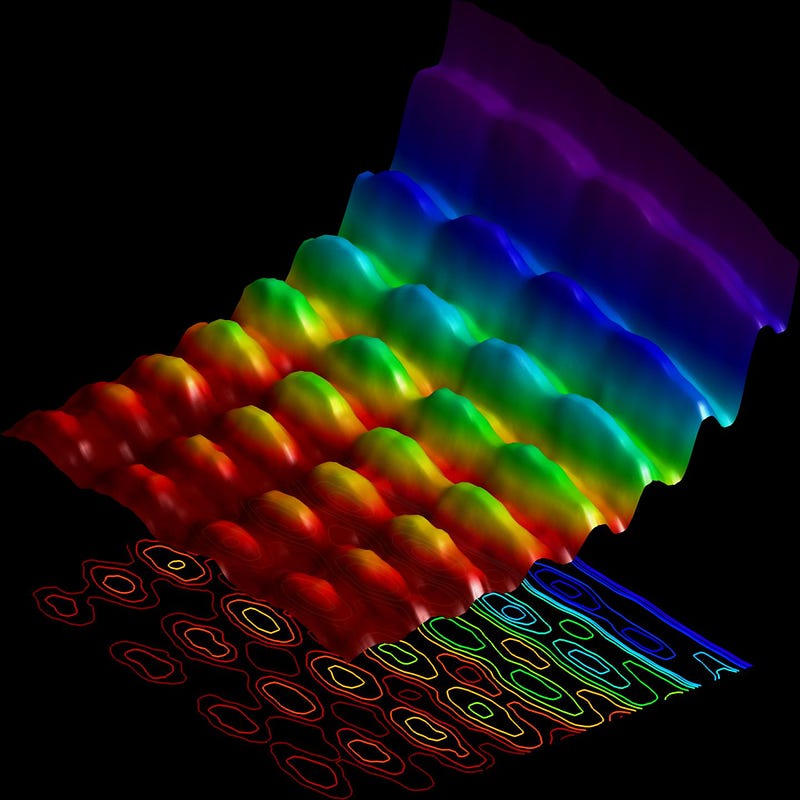
Can hidden variables provide a resolution to the mysteries of quantum physics? While we have identified several measurable variables, there might exist others that could help clarify the enigma of quantum behaviors. However, these hidden factors may not eliminate the strange phenomena observed in quantum mechanics.
Since the advent of quantum theory, scientists have grappled with the unsettling reality that our understanding of physical existence—what objects are and their inherent characteristics—appears not to be strictly predetermined. In the absence of measurement or interaction, quantum systems remain in a state of uncertainty; we can only describe their properties and probable measurement outcomes using statistical methods.
This leads us to ponder whether this uncertainty is an intrinsic aspect of nature—where reality remains indeterminate until a measurement is conducted—or if a “hidden reality” exists, one that is entirely predictable and comprehensible beneath the surface of our observations. This intriguing notion was championed by the legendary physicist Albert Einstein and is also the concern of Patreon supporter William Blair, who asks:
> “Simon Kochen and Ernst Specker logically demonstrated that hidden variables cannot exist in quantum mechanics. I have researched this, but the articles are beyond my mathematical and physical understanding. Could you clarify?”
The complexity of reality, particularly in the realm of quantum mechanics, is undeniable. To illustrate this, let's consider the Heisenberg uncertainty principle—the most renowned example of quantum indeterminacy.
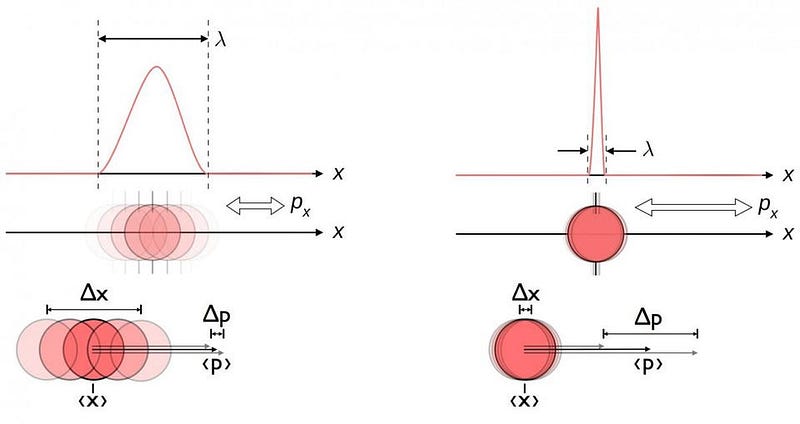
In our classical, macroscopic world, measurement issues do not arise. Whether measuring a jet, car, tennis ball, or even a speck of dust, we can accurately assess various properties and use established physical laws to predict their future states. Classical equations from Newton, Einstein, and Maxwell operate deterministically; if we know the positions and velocities of every particle, we can forecast their future states accurately, only limited by measurement precision.
However, this determinism breaks down in the quantum realm. A fundamental uncertainty governs our capacity to know multiple properties of a quantum object simultaneously. When attempting to measure, for example:
- position and momentum,
- energy and lifespan,
- spin in any two perpendicular orientations,
- or angular position and angular momentum,
you'll discover limitations on how precisely both can be known together: their product cannot be less than a fundamental value related to Planck's constant.
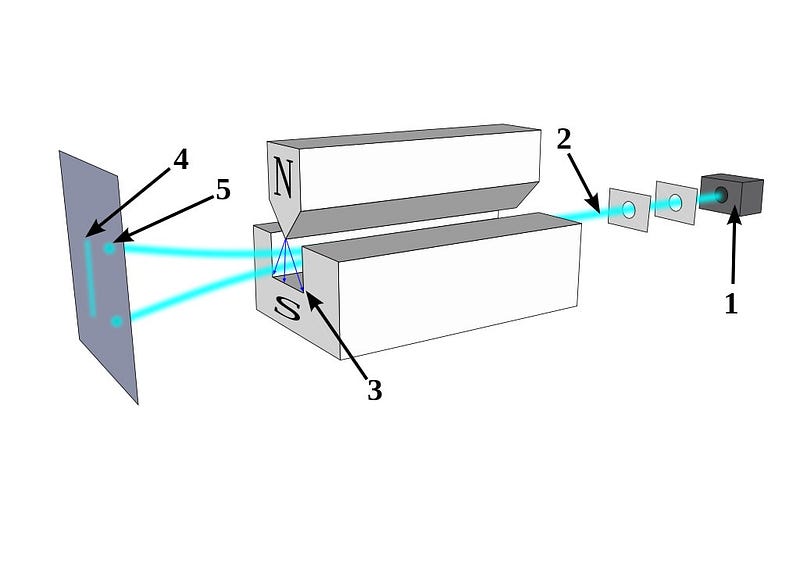
Measuring one property to high precision will invariably increase the uncertainty in the complementary property, maintaining the product above a specific threshold. The Stern-Gerlach experiment exemplifies this concept. Quantum particles, such as electrons and protons, possess an intrinsic angular momentum referred to as quantum “spin,” which, despite its name, does not imply physical rotation.
When particles like silver atoms traverse a magnetic field, they will be deflected based on their spin orientation—either positively (+½) or negatively (-½). If subsequently passed through another Stern-Gerlach apparatus aligned similarly, no further separation occurs; the particles retain their prior spin orientation.
However, if they encounter a magnetic field oriented differently, they will split again, suggesting an intrinsic uncertainty about their spin states in the new direction. When returning to the original orientation with another magnetic field, they will again exhibit the original split, indicating that measuring in a perpendicular direction has somehow erased previously known information.
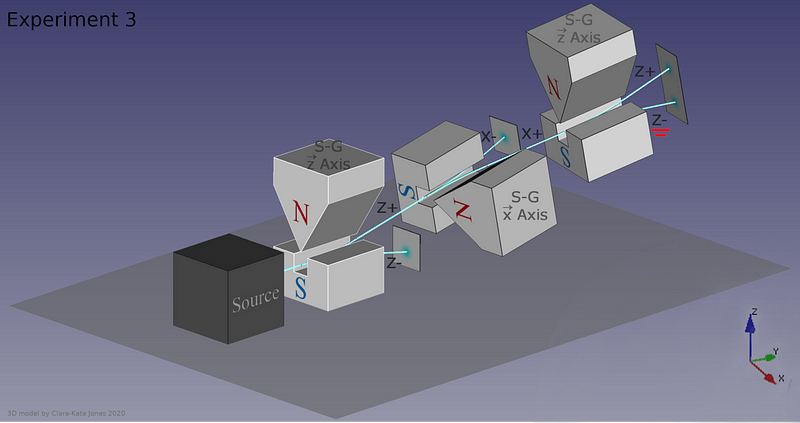
Traditionally, this is interpreted as an inherent indeterminacy in the quantum world that cannot be fully resolved. When one property is precisely determined, the uncertainty in its orthogonal counterparts must increase significantly, preventing any circumvention of the uncertainty principle; meaningful insights can only be gained through measurement.
Yet, an alternative perspective exists: the concept of hidden variables. In a scenario where hidden variables are at play, the universe could indeed be deterministic, with quantum entities possessing inherent traits that would allow precise predictions about their behavior. Still, certain governing variables remain unobservable in our current reality. If we could identify and comprehend these variables, the seemingly indeterminate behavior might be understood, revealing a less mysterious quantum universe.
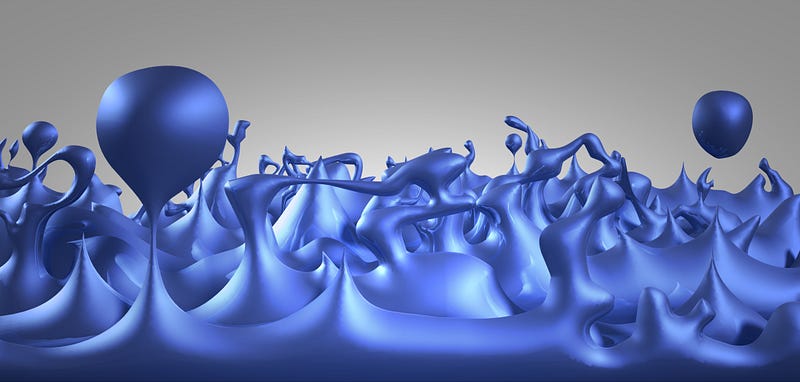
Imagining hidden variables leads to a visualization of a universe governed by dynamics we do not yet grasp; it resembles a surface of sand grains influenced by an unseen vibrating plate beneath. To the observer, the individual grains appear to fluctuate randomly, masking potential correlations that might exist due to the hidden dynamics.
This notion is intriguing, but like all scientific concepts, it must be validated through empirical evidence and experimentation.
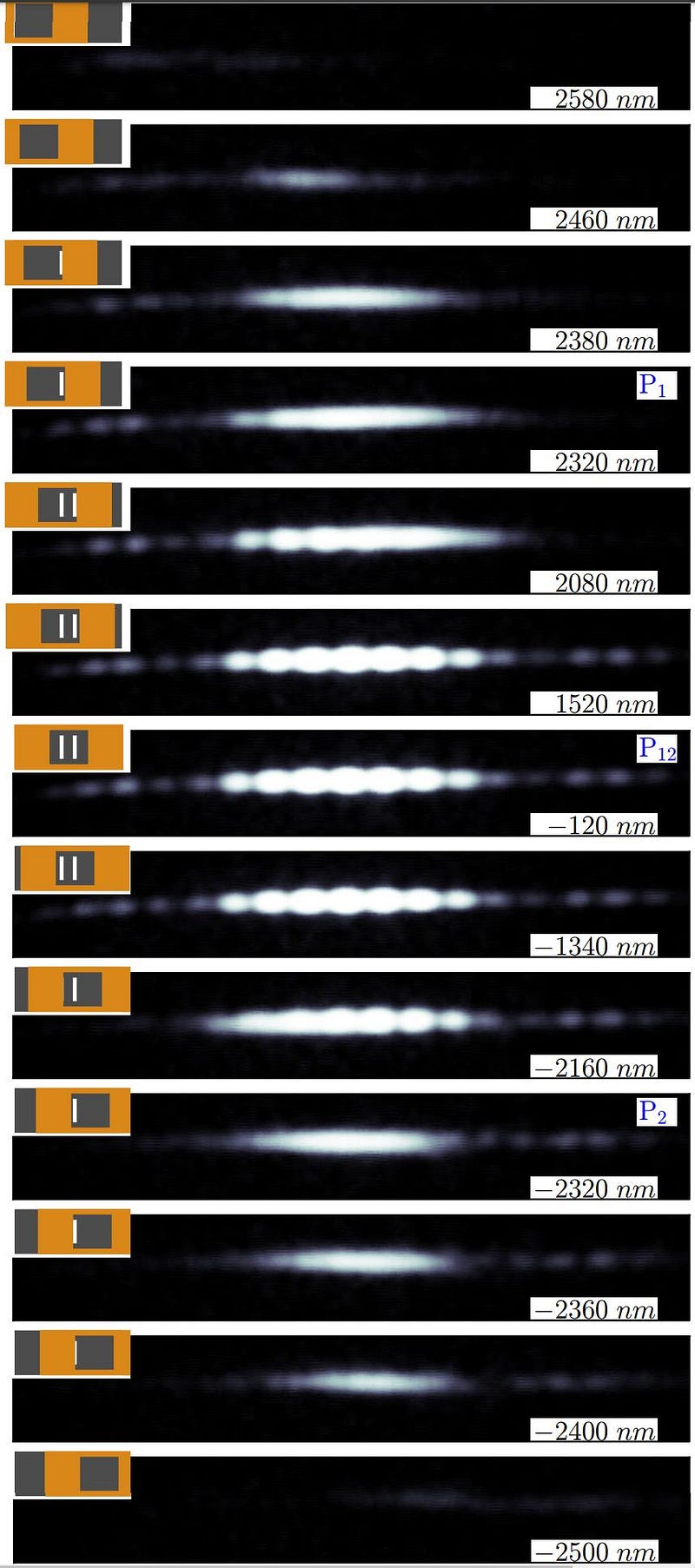
A pivotal experiment in quantum physics is the double-slit experiment. When a single quantum particle is directed at a double slit, the resulting impact pattern on a detector screen reveals fascinating outcomes over numerous trials.
The results are curious:
- Without measuring which slit the particle passes through, an interference pattern emerges, revealing areas where landing is more or less probable. This effect persists even when particles are sent one at a time, suggesting self-interference.
- Conversely, measuring which slit each particle traverses results in two distinct clumps, corresponding to each slit, obliterating the interference pattern.
To delve deeper into quantum phenomena, we can conduct delayed-choice experiments.
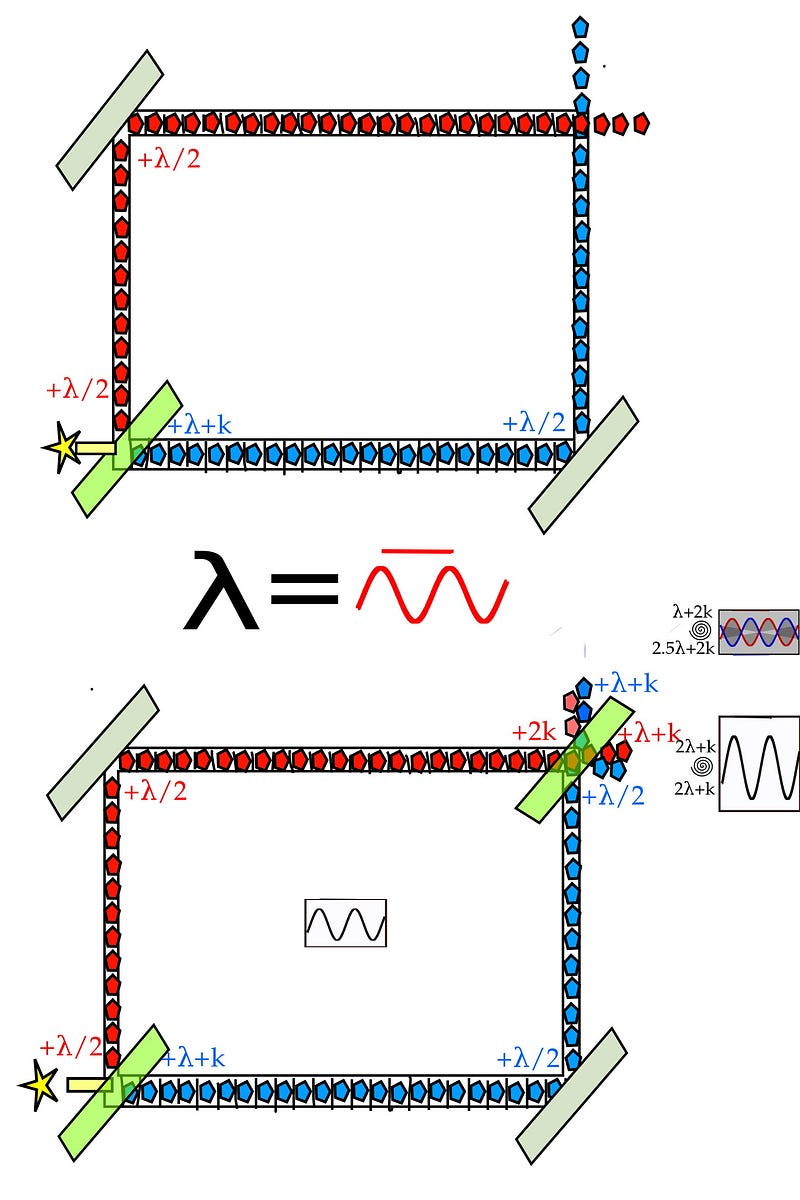
John Wheeler, a leading physicist, contemplated the peculiar nature of quanta behaving as either particles or waves. He devised experiments to observe wave-like behaviors under particle-like expectations, exemplified by passing a photon through a beam splitter into an interferometer set to either “open” or “closed” configurations.
When the configuration is “open,” individual photons are detected without generating an interference pattern. Conversely, a “closed” configuration results in observable wave-like interference.
Wheeler sought to determine if photons “knew” how to behave beforehand. By altering the experimental setup just prior to photon arrival, he aimed to capture them as either waves or particles.
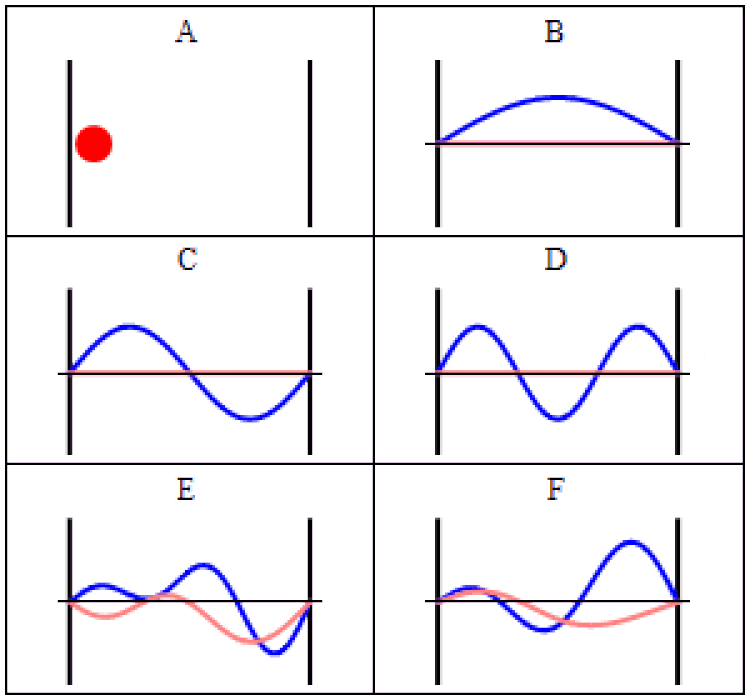
In all cases, the behavior of quanta aligns with expectations. For example, interactions during the double-slit experiment dictate particle behavior, while non-interaction leads to wave behavior. In the delayed-choice setup, the presence of a recombining device at photon arrival yields wave-like patterns; its absence results in distinct, non-interfering photons. As Niels Bohr aptly noted:
> “...it can make no difference, as regards observable effects obtainable by a definite experimental arrangement, whether our plans for constructing or handling the instruments are fixed beforehand or whether we prefer to postpone the completion of our planning until a later moment when the particle is already on its way from one instrument to another.”
Does this negate the existence of hidden variables? Not entirely. However, it imposes significant constraints on their nature. As shown by John Stewart Bell's work in 1964, any hidden variable theory that aligns with experimental results must be fundamentally nonlocal, implying that some information exchanges occur faster than light.
The Kochen-Specker theorem, established shortly after Bell's work, further complicates hidden variable theories. It suggests that measuring any quantum property is not simply revealing pre-existing values; rather, the outcomes depend on the context of simultaneous measurements. This theorem indicates that quantum contextuality—where outcomes are influenced by the measurement context—is a fundamental characteristic of quantum mechanics, making it impossible to assign values to physical quantities without disrupting their interrelationships.
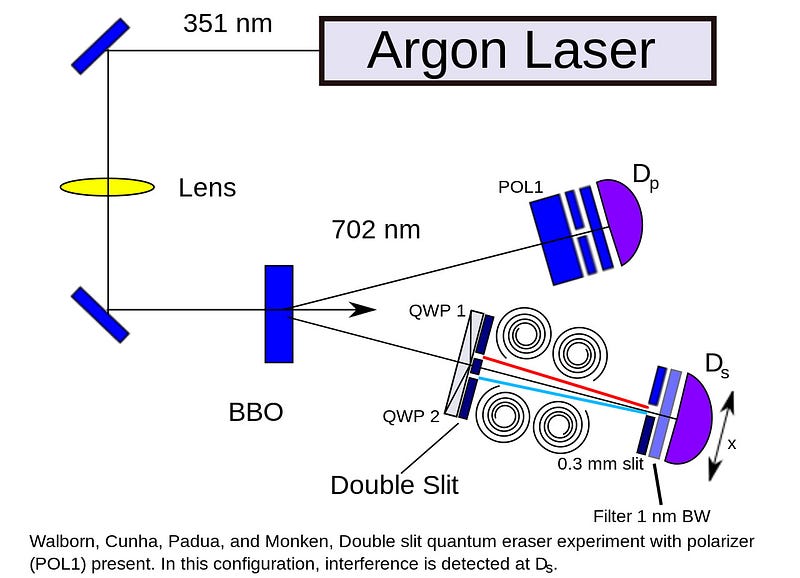
Ultimately, regardless of our confidence in logical reasoning and mathematical integrity, empirical results dictate the nature of reality. The various interpretations of quantum mechanics, while equally valid in their predictions, do not contradict the original Copenhagen interpretation. Preference for one interpretation over another often stems from personal ideology rather than empirical evidence.
If one wishes to propose hidden variables governing reality, they may do so. However, the Kochen-Specker theorem suggests that if such variables exist, they do not independently determine experimental outcomes free from the established quantum rules. This notion of quantum contextuality has emerged as a significant area of study, particularly in quantum computing, influencing advancements toward achieving quantum supremacy.
Despite our discomfort with the inherent strangeness of quantum mechanics, it's essential to recognize that alternative interpretations, including those involving hidden variables, are equally perplexing in their own ways.
For your inquiries, reach out to Ask Ethan at startswithabang at gmail dot com!
Starts With A Bang is authored by Ethan Siegel, Ph.D., writer of Beyond The Galaxy and Treknology. Pre-order his Encyclopaedia Cosmologica here!